Our Night Sky: Difference between revisions
No edit summary |
|||
(62 intermediate revisions by the same user not shown) | |||
Line 1: | Line 1: | ||
[[Image: | [[Image:Our night sky.jpg|right]] | ||
As I mentioned on the main [[The_Great_Courses|The Great Courses]] page, I like this show pretty well, and would recommend it as a good introduction to the stargazing and amateur astronomy. | As I mentioned on the main [[The_Great_Courses|The Great Courses]] page, I like this show pretty well, and would recommend it as a good introduction to the stargazing and amateur astronomy. Luckily this, pretty good, course is available on both [https://cincinnatilibrary.kanopy.com/video/our-night-sky Kanopy] and [https://www.hoopladigital.com/title/11527580 Hoopla] from my library, so it's pretty easy to watch on you computer, iOS device, and probably Roku and other platforms. | ||
= Our Night Sky = | = Our Night Sky - Professor Edward M. Murphy, Ph.D. - University of Virginia = | ||
This is the general description of the course/series. | This is the general description of the course/series. | ||
<blockquote>''For thousands of years, the star-filled sky has been a source of wonder, discovery, entertainment, and instruction. Ancient people from nearly every continent and culture wove exciting stories about the mythological figures they saw in the heavens. People also used the sun, moon, and stars for time-keeping and navigation. And careful observers throughout Europe, the Middle East, Asia, and elsewhere kept precise astronomical records, eventually paving the way for the Scientific Revolution and its remarkable discoveries about the nature of the universe.''</blockquote> | <blockquote>''For thousands of years, the star-filled sky has been a source of wonder, discovery, entertainment, and instruction. Ancient people from nearly every continent and culture wove exciting stories about the mythological figures they saw in the heavens. People also used the sun, moon, and stars for time-keeping and navigation. And careful observers throughout Europe, the Middle East, Asia, and elsewhere kept precise astronomical records, eventually paving the way for the Scientific Revolution and its remarkable discoveries about the nature of the universe.''</blockquote> | ||
Line 9: | Line 9: | ||
== Episode 1 The Constellations and Their Stars [[Image:4 | == Episode 1 The Constellations and Their Stars [[Image:4-stars.png|100px]] == | ||
This episode is really just laying out the syllabus for the course, an true overview. He then discusses, in broad terms, the origins of Constellations, and the difference between a constellation (a pattern of stars in the sky, like Ursa Major) and an asterism (the Big Dipper). He then tells us about Ptolemy’s 48 classical constellations, and the Almagest (his book and star catalog of 1,028 stars, Arabic for “the greatest” from 150 AD). Of course Ptolemy saw a geocentric theory of the universe, with the Earth in the center which dominated for 1,400 years. | |||
Next he tells us about the Greek astronomer Eudoxus (390-340BC) and how he learned about the constellations from the Egyptians before writing his Enoptron (Murrow) and Phenomena (appearances), which was later referenced by Aratus (315-245 BC) - as the original was lost to history. Next, the tells us because there were no constellations created within 36 degrees of the south celestial pole, the developers must have lived at about 36 degrees of latitude. This is south of the Greek mainland and north of the Egyptian mainland. He uses this to deduce that the original Samaritan and Babylonian cultures developed the Zodiac and the constellations. | |||
Next he tells us about other star catalogs, and brightness and color, with blue stars being comparatively hotter than red/orange stars. The are classed by letters O-30K/B-20K/A-10K/F-7K/G-6K/K-4K/M-3K (Oh Be a Fine Girl, KISS Me). Each of these class have 0-9 sub classes. | |||
He closes with distances, introducing the astronomical unit (AU), the distance of the Earth from the Sun, 1AU (150M km) and light years (5.88 trillion miles). He tells us that Voyager 1 is 19 light hours away (in 2019). Alpha Centauri, the nearest star system to us, is 4.4 light years away. Finally he tells us about sky charts and planispheres.</br> | |||
<blockquote>''Begin your study of the night sky by investigating the origin of the constellations—the traditional groupings of stars that mostly date to antiquity. The well-known constellation Orion illustrates the fascinating mix of beauty, mythology, and scientific knowledge to be found wherever you look in the heavens.''</blockquote> | <blockquote>''Begin your study of the night sky by investigating the origin of the constellations—the traditional groupings of stars that mostly date to antiquity. The well-known constellation Orion illustrates the fascinating mix of beauty, mythology, and scientific knowledge to be found wherever you look in the heavens.''</blockquote> | ||
</br> | </br> | ||
== Episode 2 Seeing and Navigating the Sky [[Image: | == Episode 2 Seeing and Navigating the Sky [[Image:4-stars.png|100px]] == | ||
This episode | This episode is another good overview, starting with what twilight means. During Civil Twilight (right after sunset), we’re told you can still read outside without artificial lighting and only the brightest stars and planets are visible. Next is nautical twilight you can see most of the celestial objects needed for navigation, but you can still see the horizon to take their altitudes. Finally is Astronomical twilight, where there is not enough light to see the horizon clearly, and the sun no longer illuminates the sky and the faintest objects are visible. Then he explains that twilight lasts longer the higher your latitude. For example, at the equator astronomical twilight ends 1 hour and 12 minutes after sunset, at higher latitudes it can last over 2 hours. We’re told that if you live in a dark sky area you can see up to 6,000 stars are bright enough to be send at night, but only about 1/2 are above the horizon. Next light pollution is explained as stray light leaking into space, and wasting energy not illuminating what we intended to see, and what a ‘full-cutoff’ light fixture is. Then we learn about night vision adaptation and red flashlights, and averted vision. | ||
Next we move on to start brightness, including apparent brightness and actual energy output (luminosity). Then we’re introduced to the inverse square law, and how light intensity drops off as the square of the distance away. The magnitude scale is then described, as defined by Hipparchus in the 2nd century BC - the brightest stars were first magnitude, and the faintest he could see were 6th magnitude. The magnitude system has been redefined so that a difference in 5 magnitude is exactly 100 times in brightness. A 1 magnitude difference is about 2.5 times, 2 magnitudes is 6x and 3 is 16x. Polaris is a 2.0, the naked eye limit is 6, binoculars are 10, and an 8” telescope in dark skies can do 14. Limiting magnitude is the dimmest star magnitude that you can see in your typical skies. A good dark sky site can see as low as magnitude 6, a typical urban site can only do as dim as magnitude 4. A city area might be as high as 3, with only about 50 stars visible. | |||
Next we learn about sky distances and the “celestial sphere”. We learn that we can estimate distances with our hands, with a finger being about 1 degree at arms length, fist being about 10 degrees, and your outstretched thumb-to-oustreteched pinky is about 20 degrees. The sun and moon are about 1/2 degree across, and the belt in Orion is about 3 degrees, and Rigel to Betelgeuse is about 18.5 degrees. Most people can only see about 1 arc minute resolution in the sky, and 1 arc second is about the size of a quarter at a distance of 3.1 miles away. Zenith is directly overhead, and he walks us through the definitions of true north (the rotational pole) and magnetic north, and how altitudes of stars and planets and their azimuths are determined, and alt-azimuth mounts for scopes. The meridian is explained as passing from the North Pole to the South Pole, passing directly overhead. The celestial equator is described as being 23 degrees above/below the horizon, to make up for the tilt in our orbital spin. | |||
Next coordinate systems are explained, with the height above the horizon being Declination, and the E/W being Right Ascension, similar to latitude and longitude. These remain constant as the object moves across the sky, they pin the object to the celestial sphere, not our observing location. The declination is relative to the celestial equator (NOT the horizon, which is tilted). Right Ascension is zero on the vernal (spring-the Sun’s south to north crossing on March 20th) equinox point - where the ecliptic crosses the celestial equator. Since Orion’s Belt is near the celestial equator, it is a good way to locate it. The equator passes just below the belt at 5H 55m. He then describes the ecliptic and the zodiac, where the sun appears to traverse the heavens. Next he tells us that planets, unlike stars, don’t twinkle, and can so be verified in the night sky. He closes with a discussion of the Messier and NGC catalogs of sky numbers.</br> | |||
<blockquote>''The naked eye is a powerful instrument—if you know how to use it. Learn the best times and conditions for observing, how to identify the positions and magnitudes of stars and planets, how the sky changes over the course of a night, how to use astronomical maps such as a planisphere, and more.''</blockquote> | <blockquote>''The naked eye is a powerful instrument—if you know how to use it. Learn the best times and conditions for observing, how to identify the positions and magnitudes of stars and planets, how the sky changes over the course of a night, how to use astronomical maps such as a planisphere, and more.''</blockquote> | ||
</br> | </br> | ||
== Episode 3 Using Binoculars and Backyard Telescopes [[Image: | == Episode 3 Using Binoculars and Backyard Telescopes [[Image:5-stars.png|100px]] == | ||
This episode was | This episode was very good, but I knew most of this already. He starts out by covering how to purchase and use a good set of binoculars. He also makes the point that some objects, such as M45 the Pleiades star cluster, look better in binoculars because the are so visually large that a wide field of view (wider than a telescope allows) is required for good viewing. He shares that aperture (or objective in a telescope) is the light opening, and determines overall image brightness, and that magnification level is completely separate. | ||
Next we learn how telescopes work, and how they typically don’t have prisms so the view is reversed left/right and upside down. We also learn about focal length, the distance down the tube where focus is achieved by the lens. He then shares that the eyepiece does the magnification, and that the objective determines the brightness again, and that generally more light collection is better. | |||
Next we learn about types of telescopes.<br/> | |||
'''Refactor''' - or types use lenses to focus light, generally at the end of a long tube. These are limited by the ability to grind big lenses, but also more importantly, the ability to support the sharp edges of a large lense under its own weight as we move it about the night sky. These typically suffer from achromatic aberrations, the fact that different wavelengths are bent different amounts. Some scopes do correct for this. | |||
'''Reflectors''' - gather light on a large mirror in back of the tube that generally reflects back from the primary mirror up the tube to a smaller angled mirror that shines the light out the side to an eye piece such as the popular ”Newtonian” type scopes. In a Cassegrain reflector the light is sent back down the tube through a small hole in the primary mirror. | |||
'''Catadioptrics''' - or combined scopes, with both mirrors and lenses.<br/> | |||
Next he describes overall magnification as the focal length of the telescope over the focal length of the eye piece. Thus the shorter the eyepiece (6mm versus 50mm) the greater the magnification - but at a cost of smaller field of view. He tells us that most people use 2030x magnification per in of objective diameter when observing planets, and up to 60x on a good steady night. We can hardly ever use over 300x because of poor seeing. Next he reminds us that star twinkling is caused by unsteadiness in the intervening atmosphere. If the stars twinkle a lot the image in the telescope will be blurry. | |||
Next we learn about field of view, or how much of the sky you can see in the eye piece. This usually means larger, more expensive lenses. Most eyepieces are quoted in apparent field of view. This is the field of view outside of a scope. The true field of view is the apparent FOV of the eye piece divided by the magnification. He then shares a cool tip. If you don’t know the FOV of the scope, find a star near the ecliptic. We know these stars move about 1 degree every 4 minutes, so if we place the star just outside the E edge of the scope and time how long it takes to clear the W edge of the scope and divide by 4, we’ll have the FOV. | |||
Eye relief is how far away from the eyepiece you can still focus the lens. Most lenses can correct for near or farsightedness, but not astigmatism, so if you have astigmatism should buy eye pieces with large reliefs. Next he describes good start scopes as being very portable and easy to maintain. Since Refractors never need to be realigned, or collimated, and cannot get dust inside the lens system, they are easy to maintain and almost never need to be cleaned. A starter scope is 2-4” objective. Above 6” diameter is quite expensive. Achromatic scopes use multiple lens to get most of the usable light focused. Apochromatic scopes use several lens to fully correct for aberration. | |||
Next he explains that Schmidt-Cassegrain and Muksutov-Cassegrain are some the most popular scopes. Then we move onto mount types, first alt-az mounts, which can cause it to be difficult to track stars. Then there is the equatorial mount that is aligned to the Earth’s rotational axis. A “GoTo” mount uses a computer to find and track objects in the sky. Equatorial mounts are really required for astrophotography, and some use “guiding” - a smaller scope that finds bright known objects and keeps them centered in the scope. Some scopes can use GPS to do their own basic alignments. Next we learn that standard eye pieces come in three sizes, .965”, 1.25”, and 2”, with the latter two being most common. They also come in focal lengths of 4mm-55mm (with the 4mm being very high magnification). It is rare that the air is steady enough (good seeing) to use more than 3-400x magnification - but also never than 60-80x per inch of aperture/objective. A ‘star diagonal’ brings the light out 90 degrees from the scope line and is really helpful for objects very close to the zenith. A Barlow lens increases the focal length, and therefore the magnification. A 2X Barlow doubles the focal length of the scope and doubles the magnification of any eyepiece that you put in the scope - it’s really important to buy a good Barlow. It also preservers the eye relief for those with glasses, so you can use a lower power eyepiece with a Barlow to get good magnification with a lower power eyepiece with good relief. We finish learning about filters. Filters thread into the bottom of standard eyepieces. First is the Moon filter that cuts the extreme brightness of the Moon when it’s near full. Some are adjustable even. Next is the light pollution filter, which dampens light in the manmade wavelengths, which is especially useful when looking at nebula. </br> | |||
<blockquote>''here are many choices when selecting binoculars or a telescope. Learn what to look for in light-gathering power, optical design, magnification, mounts, and other features. Professor Murphy also suggests several tips for getting the best observing experience out of your equipment.''</blockquote> | <blockquote>''here are many choices when selecting binoculars or a telescope. Learn what to look for in light-gathering power, optical design, magnification, mounts, and other features. Professor Murphy also suggests several tips for getting the best observing experience out of your equipment.''</blockquote> | ||
</br> | </br> | ||
Line 27: | Line 58: | ||
== Episode 4 Observing the Moon and the Sun [[Image:4-stars.png|100px]] == | == Episode 4 Observing the Moon and the Sun [[Image:4-stars.png|100px]] == | ||
[[File:Moon phases.jpg|thumb|left]] | |||
In this episode we first learn about how the Moon appears bigger when it’s on the horizon. Then we learn that the full moon always rises at sunset, which leads us to the Phases of the Moon. First we learn that “new moon” is completely black, directly to the sunward side of the Earth where we see the un-illuminated side. Then we find the crescent moon. It is 29.5 days from new moon to new moon. The first 2 weeks the moon rises at sunset, the next 2 it sets at sunrise. Waxing moons are lit from the right and waning at the left (in the northern hemisphere). | |||
Next we learn that, although the moon rotates, the same side always faces the Earth (it is tidally locked), and the “dark” side looks very different than the side facing the Earth (which does actually get illuminated). This means it rotates on it’s axis in the time it takes to orbit the earth (once a month). Next we learn that it is best to view the moon near the quarter, and view along the ‘terminator’, or the day/night line, since the shadows bring out a lot of additional detail. We then learn that the highlands are 4.1-4.4 billion years old, and the maria (‘seas’, or the dark spots where molten rock filled in after heavy asteroids hit) are 3-3.8 billion years old. The Maria cover about 31% of the near side of the Moon. Then we learn that the craters about 10X bigger than the objects that created them. Next we learn that the “rays” are ejected material from impacts landing on the older, darker surface of the Moon so they appear brighter. | |||
Next we learn about the Sun’s yearly trip through the stars of the Zodiac constellations. Then we learn that the seasons on Earth are related to our 23.5 degree tilt. In summer (in either hemisphere) that the tilt is towards the sun, and winter the tilt is away from the sun. The equinoxes are when the Sun crosses the equator, and the solstices are when the sun stops moving north or south, and starts heading the other way. The Tropics are mark where, on the solstices, the sun is directly overhead, and the sun is overhead of the equator on the equinoxes. Next we learn that the sun never rises within 23.5 degrees of the poles in mid winter, and never sets within that range in summer - the Arctic/Antarctic circle.</br> | |||
<blockquote>''Charting the motions and changes of the sun and moon may be humankind's oldest astronomical activity. Discover how both objects offer rich opportunities for study. Also learn the precautions to take when observing the sun, which is the only star that can be seen up close and in detail.''</blockquote> | <blockquote>''Charting the motions and changes of the sun and moon may be humankind's oldest astronomical activity. Discover how both objects offer rich opportunities for study. Also learn the precautions to take when observing the sun, which is the only star that can be seen up close and in detail.''</blockquote> | ||
</br> | </br> | ||
== Episode 5 Observing the Planets with a Telescope [[Image:4-stars.png|100px]] == | == Episode 5 Observing the Planets with a Telescope [[Image:4-stars.png|100px]] == | ||
This episode starts with sharing that both Mercury and Venus both go through phases, just like the Moon does. Then we learn that there are 3 types of planets, the inner 4 (Mercury, Venus, Earth, and Mars) which are hard, rocky planets. Then we have the gas giants (Jupiter, Saturn, Uranus, and Neptune). Then we have the icy bodies, Pluto and Eris in the Kupier Belt. | |||
Since Mercury is closer to the Sun than us, we can never see it very far from the Sun, so it is always seen close to dawn or dusk (best at it’s greatest elongation). The same is true of Venus, although it gets a little further away, but it is clearly larger when it is closer to us. Mercury is 38% the diameter of the Earth and 5.5% the mass, and orbits at .4 AU in 88 days. It has no atmosphere and looks muck like the Moon, but this is not visible from Earth with typical telescopes. It’s size varies from 5 to 13 arc seconds in the sky, depending on where it is in it’s orbit. Mercury is never more than 28 degrees from the Sun, so it is always low to the horizon, so trees and buildings, and turbulence are issues. | |||
Venus is the hottest planet in the solar system, even though it’s twice as far away from the Sun as Mercury. It’s 95% the diameter of Earth and orbits in 225 days a .72 AU (so it gets closer to Earth than any other planet), and 82% the mass but the atmosphere is 92x that of Earth. The average surface temp is 870F. It’s as small as 10 arc second, and gets as big as 65 arc seconds on closest approach, and is the third brightest object in our sky. It reaches greatest elongation of 45-47 degrees from the sun, and is visible for many weeks in the sky. | |||
Mars is one of the most popular objects to look at, but is difficult to see features on because it is so small, only 53% the diameter of the earth and 11% the mass, orbiting in 687 days (1.88 years) at 1.5 AU. To see detail on Mars it must be viewed at Opposition, when it’s closest to the Earth, which only happens every 26 months. Mars’ orbit the most elliptical orbit of the planets, so sometimes Mars is much closer (perihelic-56 million km) opposition, and much further away at aphelic (no closer than 101 million km) opposition. Can be as big as 25 arc seconds across at perihelic opposition, at aphelic it’s 14 arc seconds, and can be as small as 4 arc second when on the far side of the Sun. Viewing is good for a month on either side of opposition. Upcoming oppositions are October 13, 2020 and December 8 2022. Mars rotates every 24 hours and 40 minutes on its axis. The atmosphere is on .7% that of Earth, and mostly CO2. Mars has 2 small moon, Phobos (fear) and Deimos (panic) and are 22 and 12 km, respectively, and are probably captured satellites. Blue and green filters make the polar caps more visible, and red/yellow make the dust storms and dark patches more distinct. #15 deep yellow, #25 red, #58 green and #80a blue are common. | |||
The gas giants are mostly hydrogen and helium. Jupiter is 11.2x the diameter and 318x the mass of the Earth (greater than the rest of the planets put together). It orbits in 12 years at 5.2 AU. At opposition Jupiter can be 44-50 arc seconds across, and never small than 30, and comes to opposition ever 13 months, and is good for about 2 months on either side of opposition. You’ll easily see the cloud bands and the 4 big moons (Is, Ganymede, Europa, and Callisto). Jupiter has dark belts and bright zones. She rotates in 9.8 hours. The red spot is 2-3x the size of the Earth. Io orbits in 1.8 days, Callisto requires 17 days. | |||
Saturn is spectacular in a scope. It’s 9.5x the diameter and orbits 9.5 AU from Earth, and comes to opposition every 54 weeks when it is 18-21 arc seconds in size. It is well placed for a few months before and after opposition. The rings span more than 270,000 km, but are tens of meter thick. The rings are around the equator, but are tilted about 27 degrees with respect to the orbit. They appear edge on about every 15 years, and it orbits the sun about every 30 years. The rings are number A-C, with A being the outermost. Saturn has over 60 moons, the largest is Titan, and is the only moon in the solar system with a substantial atmosphere. | |||
Uranus 4x diameter of the Earth and orbits at 19.2 AU in 84 years. At it’s largest it’s only 4 arc seconds across, and it’s axis is tipped 98 degrees to it’s orbital axis. | |||
Neptune is a near twin to Uranus, and is 3.8x the diameter of Earth and orbits at 30AU, taking 165 years to orbit the Sun. At it’s best it’s only 2 arc seconds across. | |||
Pluto is the largest of the Kuiper Belt Objects. | |||
</br> | </br> | ||
<blockquote>''The rings of Saturn, the bands of Jupiter, the phases of Venus, the polar caps of Mars—these and other planetary features are visible through a small telescope. Learn when viewing opportunities arise for each of the planets and what sights await the dedicated observer." and…''</blockquote> | <blockquote>''The rings of Saturn, the bands of Jupiter, the phases of Venus, the polar caps of Mars—these and other planetary features are visible through a small telescope. Learn when viewing opportunities arise for each of the planets and what sights await the dedicated observer." and…''</blockquote> | ||
</br> | </br> | ||
== Episode 6 Meteor Showers, Comets, Eclipses, and More [[Image:3-stars.png|100px]] == | == Episode 6 Meteor Showers, Comets, Eclipses, and More [[Image:3.5-stars.png|100px]] == | ||
Interesting, but to me, not as interesting as some of the other episodes. | Interesting, but to me, not as interesting as some of the other episodes. First we learn that many meteor showers and comets are within traveling distance of us seeing, and that in fact there are often a couple of faint comets viewable with a small to medium telescope most of the time. Next we learn that comets orbit on highly elliptical orbits, often coming closer than Mercury and Venus to the Sun before heading back out into the solar system - and that most of the brightest have periods in the thousands of years! The comet tail can be millions of miles long, and always points away from the sun (due to the solar wind). | ||
Meteor showers are caused when the Earth travels through debris left behind by a comet or asteroid (and minor planets). On any clear night you can expect to see a few to maybe 10 meteoroids per hour. They are meteoroids until they hit our atmosphere, at which point they become meteors, and anything that hits the ground is a meteorite. Most meteoroids are grain to pebble sized. They enter at 7-45 mi/sec, and the glow is caused by heated gas (air) around the meteor. A very bright meteor is a Fireball. The dense trail of meteoroids left by a comet can create meteor showers. These appear to come from one location in the sky called the Radiant, and are named for the constellation that they radiate from. A measure of how many meteors you will see in an hour is the Zenith Hourly rate, which is how many would see in an hour if they are near the zenith where there is little interference. All showers are generally 1 day before or after the published "best" day, depending on our orbit and your viewing location. A bright moon with obscure most meteors, and the field of view of a telescope or binoculars are too small to find meteors, and are typically best from midnight to noon, when you're looking in the direction of travel of the Earth's orbit. | |||
Total eclipses, like comets, have long had negatively connotations in human history. It's a happy coincidence that the moon and the sun are both 1/2 degree across in our sky, so that total eclipses are possible. The umbra is the dark part of the shadow of the moon, and the penumbra is where it is not totally covered. There are ways to safely look at the sun, but must be strictly followed. Planets and bright stars appear in the sky, and there is light on all horizon directions. Next we’re told about annular eclipses, and how the Sun and Moon’s apparent sizes change over time, depending on how close they are, and times the Moon is simply not big enough to fully cover the sun. A total eclipse is at best 169 miles across and can last at most 7 minutes due to the moons speed (1,000 MPH). | |||
Conversely we learn that total lunar eclipses are visible from the entire night side of the Earth, and do not happen every month because the Moon’s orbit is inclined at 5%. This inclination makes the Moon miss the Earth’s umbra on most full moons. They only happen twice a year, during ‘eclipse season’ when the Moon is passing from above or below the ecliptic to the other side, just as the sun does with changing seasons. This is where the ecliptic gets its name. Next we learn about penumbra, partial, and total eclipses. A total eclipse of the Moon can last for 2 hours, so in 3-4 hours we go from full moon to partial then full eclipse, and back to full. Because light bends around the Earth usually the eclipsed Moon looks reddish. There are 2-4 lunar eclipses every year, with a total eclipse every 18 months or so. | |||
Finally we learn that Mercury (7%) and Venus (3.4%) also cast shadows onto Sun. Mercury requires a telescope. This happens 13 or 14 times a century. Transit of Venus happen in pairs, separated by 8 years, but the transits only happen every 105.5 or 121.5 years, in January and June and last about 6 hours. The next topic are halos around the moon or sun, which are high cirrus clouds filled with hexagonal ice crystals, and the light gets bent by 22 degrees or more creating the halo (sun dogs). Then we learn that satellites can been seen a few hours after sunset or before sunrise, when they are still above the horizon and in the sun. | |||
</br> | </br> | ||
<blockquote>''Explore a variety of special phenomena that are among the wonders of the sky. Some, like bright meteors, aurora, and many comets, are largely unpredictable. Others, like eclipses and annual meteor showers, occur at well-known times—although it may require a special trip to see them.''</blockquote> | <blockquote>''Explore a variety of special phenomena that are among the wonders of the sky. Some, like bright meteors, aurora, and many comets, are largely unpredictable. Others, like eclipses and annual meteor showers, occur at well-known times—although it may require a special trip to see them.''</blockquote> | ||
</br> | </br> | ||
== Episode 7 The Northern Sky and the North Celestial Pole [[Image:3 | == Episode 7 The Northern Sky and the North Celestial Pole [[Image:3-stars.png|100px]] == | ||
This episode starts out with Ursa Major, which includes the Big Dipper. We learn that the two stars at the end of the dipper point us to the North Star Polaris (28 degrees away, a little further than your outstretched fingers plus your fist) - and it’s the end star of the Little Dipper. The W of the Cassiopeia can be found by drawing a line from the Big Dipper through Polaris to the middle star of Cassiopeia, which lies int he Milky Way. The stars appear to move on a ‘celestial sphere’, and the stars appear to rotate counterclockwise. Next we learn about Southern Hemisphere stars that never rise for mid northern latitudes like the US and Europe, such as the Southern Cross. You never see any part of the cross above 33 degrees, and you must be below 27 degrees to see all of the cross. Next we learn that the altitude of Polaris is the same as our latitude. | |||
Next we learn about the wobble of the Earth, or precession, which takes about 26,000 years. This means that the north celestial pole moves through Vega and Thuban. Since Right Ascension is defined as zero where the vernal equinox happens in the stars of the zodiac, and this is moving with precession, star catalogs must publish the year, or epoch, that they are good for as the RA is constantly changing. | |||
</br> | </br> | ||
<blockquote>''Embarking on the second half of the course in which you systematically tour the entire sky, study two constellations that are continuously in view from the Northern Hemisphere: Ursa Major and Cassiopeia. Also explore the slowly shifting position of true north in the sky.''</blockquote> | <blockquote>''Embarking on the second half of the course in which you systematically tour the entire sky, study two constellations that are continuously in view from the Northern Hemisphere: Ursa Major and Cassiopeia. Also explore the slowly shifting position of true north in the sky.''</blockquote> | ||
Line 50: | Line 114: | ||
== Episode 8 The Fall Sky [[Image:3-stars.png|100px]] == | == Episode 8 The Fall Sky [[Image:3-stars.png|100px]] == | ||
Again, interesting, but | Again, interesting, although I’m not a huge fan of the ‘sky tour’ episodes. As such I’m not going to retell the constellation myths, but they are entertaining. We do learn about binary star pairs and how exoplanets are discovered, such as the 3 around upsilon Andromeda. Then we learn about the Andromeda galaxy at 2.5 million light years away it is the furthest object you can see with the naked eye. It contains about 400 billion stars, nearly double the Milky Way although we nearly as massive, and is the closest example of spiral galaxy. It’s tipped about 77 degrees to our line of sight, so it’s nearly edge on. It’s 150,000 LY across, about 1.5 times the Milky Way. Andromeda is the largest in our local group (the Milky Way, M31 Andromeda, M33, and about 30 smaller ones). Andromeda is approaching at 75 miles/sec, and we expect them to collide in about 3 billion years. M31, the Andromeda galaxy, is 8-10 times the length of the diameter of the full moon. M31 has 2 companions, M110 and M32, both dwarf elliptical galaxies. | ||
Just south of Andromeda is the Triangulum, which features M33, the Triangulum Galaxy which is the smallest of our 3 spiral galaxies in our Local Group, but we see it face on and is often called the Pinwheel galaxy. It is 1/2 the diameter and 1/7 the mass of the Milky Way. | |||
</br> | </br> | ||
<blockquote>''Navigate your way around the autumn sky from the Northern Hemisphere, discovering how the classical myth of Andromeda ties together the stories of the nearby constellations of Cassiopeia, Perseus, Cepheus, Pegasus, and Cetus. The sights include the Andromeda galaxy, the nearest large galaxy to our own.''</blockquote> | <blockquote>''Navigate your way around the autumn sky from the Northern Hemisphere, discovering how the classical myth of Andromeda ties together the stories of the nearby constellations of Cassiopeia, Perseus, Cepheus, Pegasus, and Cetus. The sights include the Andromeda galaxy, the nearest large galaxy to our own.''</blockquote> | ||
</br> | </br> | ||
== Episode 9 The Winter Sky [[Image:3 | == Episode 9 The Winter Sky [[Image:3-stars.png|100px]] == | ||
Another pretty good episode, | Another pretty good episode, we start by learning about Orion and the constellations he can point us to. Next we learn about Betelgeuse in Orion’s armpit, a Class M super giant star, 640 LY away from our Sun, and is the biggest star of any kind within a few thousand light years of the sun. If it were in place of our sun, it’s radius of 5AU would consume all of the inner solar system and out to Jupiter. It is one of the very few stars that are close enough that we can see surface features. It is a red giant that is beginning to die, having used up the majority of it’s hydrogen. When it dies it will be brighter than the Moon and visible in the daytime for a few months. In the belt is Orion’s Nebula, which looks like a fuzzy greenish white cloud with 4 new born stars (the Trapezium) buried within. The nebula is 1530 LY away, making it the closest large star forming region (all these stars are under 1 million years old). Also on Orion’s Belt is the Horsehead nebula, a smaller nebulosity. | ||
Canis Major and Minor, Orion’s hunting dogs, including Sirius - a A1 class star only 8.6 LY away, one of the closest star systems, and the brightest in our skies. Next we move to Taurus the Bull and the Pleiades. Aldebaran is the largest star in Taurus, which means ‘the follower’ because it follows Taurus. It’s a K3 only 67 LY from the Sun. | |||
Open Clusters typically have 50-100 stars in the plain of the Milky Way with young, hot, blue stars. Globular clusters are bigger, having a 10,000 to a million and are not on the plane, but typically surrounding it in a halo near the center. All the stars in a cluster are the same distance away from us, and have the same composition. The Pleiades (M45) is an open cluster, ‘the Seven Sisters’. Folklore has it that it’s time to plant when the Sister become visible, and to harvest when the set for the winter.Between Perseus and Cassiopeia is the double cluster NGC 884/869, the open clusters. A low power, wide field eye piece, will fit both clusters. Another nearby cluster are the Hyades within the face of the Bull. The Hyades are one of the most clusters for judging distances and luminosities of other stars, and are just 151 LY away. Also in Taurus is M1, the Crab Nebula, and exploded star. | |||
Next we learn about Castor and Pollux, the heads of the Gemini twins. Near the twins feet is M35, an open cluster. In Cancer we find M44, the Beehive cluster (or Praesepe). The Tropic of Cancer was named for this constellation as the Sun used to be in Cancer on the solstice, but precession has since moved it. The Beehive is a good binocular target, about 550 LY away, with about 1,500 stars about 600 million years old. Within Auriga the charioteer is Capella, but it contains M36/37/M38, all good small telescope objects. | |||
</br> | </br> | ||
<blockquote>''Continuing your focus on the constellations of the Northern Hemisphere, survey the magnificent winter sky, dominated by Orion. "Star hop" around the region, which includes a wealth of interesting stars, globular clusters, nebulae, and other features, especially the Orion Nebula—the finest nebula in the northern sky—and the Pleiades and Hyades star clusters in Taurus.''</blockquote> | <blockquote>''Continuing your focus on the constellations of the Northern Hemisphere, survey the magnificent winter sky, dominated by Orion. "Star hop" around the region, which includes a wealth of interesting stars, globular clusters, nebulae, and other features, especially the Orion Nebula—the finest nebula in the northern sky—and the Pleiades and Hyades star clusters in Taurus.''</blockquote> | ||
Line 62: | Line 134: | ||
== Episode 10 The Spring Sky [[Image:3-stars.png|100px]] == | == Episode 10 The Spring Sky [[Image:3-stars.png|100px]] == | ||
Pretty good, but constellation history is covered everywhere, so a lot of this wasn't really new to me. | Pretty good, but constellation history is covered everywhere, so a lot of this wasn't really new to me. Ursa Major, Virgo, and Bootes are the main constellations. Leo is also in the sky, and Leo contains Regulus about 79 LY away, and Algieba which is a double star (126 LY away). Leo also contains 2 triplets of galaxies about 26 million LY away M95/96/105 is the first and M65/66/NGC3628 the second. | ||
There is in this part of the sky, near Virgo, is the Realm of galaxies. In the diamond of Virgo, between Arcturus, Cor Carolina, Denebola, and Spica, on the right hand side between Virgo and Coma Berenice are a hundred or more galaxies, about 60 million light years away and 14 million wide, including M84/86/87. Many of these are elliptical galaxies which only have old yellow, orange, and red stars, having used up their gas long ago. Within M87 is a supermassive black hole with a large accretion disk and large jets of material being expelled close to the speed of light. This makes it (Virgo A) one of the brightest radio targets. | |||
In the southern part of Virgo is a nearly edge-on spiral galaxy, M104 the Sombrero Galaxy. | |||
</br> | </br> | ||
<blockquote>''The spring sky opens the view into intergalactic space perpendicular to the plane of the Milky Way. Among the objects visible are the immensely rich galaxy clusters in Virgo and Coma Berenices, which are many millions of light-years distant and can be seen with small and moderate telescopes.''</blockquote> | <blockquote>''The spring sky opens the view into intergalactic space perpendicular to the plane of the Milky Way. Among the objects visible are the immensely rich galaxy clusters in Virgo and Coma Berenices, which are many millions of light-years distant and can be seen with small and moderate telescopes.''</blockquote> | ||
Line 68: | Line 144: | ||
== Episode 11 The Summer Sky [[Image:3-stars.png|100px]] == | == Episode 11 The Summer Sky [[Image:3-stars.png|100px]] == | ||
Pretty good, but again, like | Pretty good, but again, a sky tour. We start by learning how to use Ursa Major to find the Summer Triangle and Vega (and the Northern Cross). Then we learn about Epislon Lyrae, the double double star. Apparently those with excellent vision can detect this is a double star, but with about 100X-125X magnification shows that each star is actually a double. Then ere is the Ring Nebula (M57), which requires a scope, but with 125-200X magnification you can see what looks like a smoke ring. This is a planetary nebula, the final stage of a star, much lower power than a supernova, and this is what we expect to happen to the Sun. | ||
At the top of the Summer Triangle is Deneb, 1,425 LY away, a blue super giant 110x the radius and 54,000X more luminous than the Sun and in one night it puts out as much energy as the sun in 300 years. Then we look at Albiero, the most beautiful double in the sky with a 3.1 yellow K giant and 5.1 hot blue B8 star 34 arc seconds apart (pretty far for binaries). Within Hercules is M13, the great globular cluster, about 25,000 LY away 150 LY across, and contains about few hundred thousand stars. Also in Hercules is M92 is another globular cluster in the northern part of the constellations. | |||
Near Antares in Scorpius is the globular cluster M4, too close to Antares to see with the naked eye. Also in Scorpius are M6 (the Butterfly) and M7 (Ptolemy’s cluster), open clusters. M6 has about 50 stars, M7 appears as a bright knot, and is best viewed with binoculars. Just outside of Scorpius is 18 Scorpii, a star very similar to our Sun, 45.7 LY away. | |||
Ophiuchus is a huge constellations to the north of Scorpius. Nearby is Sagittarius, which is on the plane of the Milky Way, so it is very rich, but it does not rise very high in mid northern latitudes. Here is M8, the Lagoon Nebula, which looks split in half by a lagoon. Just north and west is M20, the Trifid nebula. Also M17 (Omega or Swan) and M16 (Eagle nebula) are here. | |||
</br> | </br> | ||
<blockquote>''Arching high overhead in the summer sky is the Milky Way, which is the plane of our galaxy seen from the inside. Tour this densely packed region of stars of all types, from dusty regions of star birth to the exquisite shells of dying stars. Here, a useful orienting feature is the Summer Triangle.''</blockquote> | <blockquote>''Arching high overhead in the summer sky is the Milky Way, which is the plane of our galaxy seen from the inside. Tour this densely packed region of stars of all types, from dusty regions of star birth to the exquisite shells of dying stars. Here, a useful orienting feature is the Summer Triangle.''</blockquote> | ||
Line 74: | Line 156: | ||
== Episode 12 The Southern Sky and the Milky Way [[Image:3-stars.png|100px]] == | == Episode 12 The Southern Sky and the Milky Way [[Image:3-stars.png|100px]] == | ||
A sky tour and of a sky I’ll almost never see. Meh. Not that it isn’t well done, but just not much use to me. First we learn about Crux, the Southern Cross, not to be confused with the false cross. It is on the flags of Australia, New Zealand, Papua New Guinea, and Brazil. Acrux is the brightest star at the bottom of the cross. The coal sack nebula is just south and west of the Cross in the Milky Way. | |||
Alpha Centauri is the third brightest star in the sky, and is in Centaurus, and is 4.36 LY away, and is the closest star system to our Sun, and is a binary. A is a G2 Star just like our sun, and the partner is a K2 cooler star. Also nearby is Proxima Centauri, only 4.22 LY, the closest of all. It also contains the globular Cluster Omega Centauri. Also the galaxy Centaurus A, NGC5128, visible in binoculars with a dark dust plane, but otherwise looks like an elliptical. | |||
Near the Small Magellanic Cloud is 47 Tuncanae, a globular cluster. In late spring and early summer the large Magallanic cloud are both visible, companion galaxies to our Milky Way. They are both irregular galaxies. The tarantula nebula is also nearby. Canopus, or Alpha Carinae, is in the Argo nearly due south of Sirius and is a rare Class F star. The Carina Nebula is nearby at 75 LY and is 4X larger than the Orion Nebula. In here is Eta Carina, which is a huge and unstable star. | |||
</br> | </br> | ||
<blockquote>''In this final lecture, travel to the Southern Hemisphere for sky views inaccessible from northern latitudes. Discover the famous Southern Cross, the Large and Small Magellanic Clouds, and a spectacular panorama of the Milky Way—along with new myths and stories that add a human dimension to our marvelous night sky.''</blockquote> | <blockquote>''In this final lecture, travel to the Southern Hemisphere for sky views inaccessible from northern latitudes. Discover the famous Southern Cross, the Large and Small Magellanic Clouds, and a spectacular panorama of the Milky Way—along with new myths and stories that add a human dimension to our marvelous night sky.''</blockquote> | ||
</br> | </br> |
Latest revision as of 12:23, 21 April 2019
As I mentioned on the main The Great Courses page, I like this show pretty well, and would recommend it as a good introduction to the stargazing and amateur astronomy. Luckily this, pretty good, course is available on both Kanopy and Hoopla from my library, so it's pretty easy to watch on you computer, iOS device, and probably Roku and other platforms.
Our Night Sky - Professor Edward M. Murphy, Ph.D. - University of Virginia
This is the general description of the course/series.
For thousands of years, the star-filled sky has been a source of wonder, discovery, entertainment, and instruction. Ancient people from nearly every continent and culture wove exciting stories about the mythological figures they saw in the heavens. People also used the sun, moon, and stars for time-keeping and navigation. And careful observers throughout Europe, the Middle East, Asia, and elsewhere kept precise astronomical records, eventually paving the way for the Scientific Revolution and its remarkable discoveries about the nature of the universe.
Episode 1 The Constellations and Their Stars 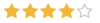
This episode is really just laying out the syllabus for the course, an true overview. He then discusses, in broad terms, the origins of Constellations, and the difference between a constellation (a pattern of stars in the sky, like Ursa Major) and an asterism (the Big Dipper). He then tells us about Ptolemy’s 48 classical constellations, and the Almagest (his book and star catalog of 1,028 stars, Arabic for “the greatest” from 150 AD). Of course Ptolemy saw a geocentric theory of the universe, with the Earth in the center which dominated for 1,400 years.
Next he tells us about the Greek astronomer Eudoxus (390-340BC) and how he learned about the constellations from the Egyptians before writing his Enoptron (Murrow) and Phenomena (appearances), which was later referenced by Aratus (315-245 BC) - as the original was lost to history. Next, the tells us because there were no constellations created within 36 degrees of the south celestial pole, the developers must have lived at about 36 degrees of latitude. This is south of the Greek mainland and north of the Egyptian mainland. He uses this to deduce that the original Samaritan and Babylonian cultures developed the Zodiac and the constellations.
Next he tells us about other star catalogs, and brightness and color, with blue stars being comparatively hotter than red/orange stars. The are classed by letters O-30K/B-20K/A-10K/F-7K/G-6K/K-4K/M-3K (Oh Be a Fine Girl, KISS Me). Each of these class have 0-9 sub classes.
He closes with distances, introducing the astronomical unit (AU), the distance of the Earth from the Sun, 1AU (150M km) and light years (5.88 trillion miles). He tells us that Voyager 1 is 19 light hours away (in 2019). Alpha Centauri, the nearest star system to us, is 4.4 light years away. Finally he tells us about sky charts and planispheres.
Begin your study of the night sky by investigating the origin of the constellations—the traditional groupings of stars that mostly date to antiquity. The well-known constellation Orion illustrates the fascinating mix of beauty, mythology, and scientific knowledge to be found wherever you look in the heavens.
This episode is another good overview, starting with what twilight means. During Civil Twilight (right after sunset), we’re told you can still read outside without artificial lighting and only the brightest stars and planets are visible. Next is nautical twilight you can see most of the celestial objects needed for navigation, but you can still see the horizon to take their altitudes. Finally is Astronomical twilight, where there is not enough light to see the horizon clearly, and the sun no longer illuminates the sky and the faintest objects are visible. Then he explains that twilight lasts longer the higher your latitude. For example, at the equator astronomical twilight ends 1 hour and 12 minutes after sunset, at higher latitudes it can last over 2 hours. We’re told that if you live in a dark sky area you can see up to 6,000 stars are bright enough to be send at night, but only about 1/2 are above the horizon. Next light pollution is explained as stray light leaking into space, and wasting energy not illuminating what we intended to see, and what a ‘full-cutoff’ light fixture is. Then we learn about night vision adaptation and red flashlights, and averted vision.
Next we move on to start brightness, including apparent brightness and actual energy output (luminosity). Then we’re introduced to the inverse square law, and how light intensity drops off as the square of the distance away. The magnitude scale is then described, as defined by Hipparchus in the 2nd century BC - the brightest stars were first magnitude, and the faintest he could see were 6th magnitude. The magnitude system has been redefined so that a difference in 5 magnitude is exactly 100 times in brightness. A 1 magnitude difference is about 2.5 times, 2 magnitudes is 6x and 3 is 16x. Polaris is a 2.0, the naked eye limit is 6, binoculars are 10, and an 8” telescope in dark skies can do 14. Limiting magnitude is the dimmest star magnitude that you can see in your typical skies. A good dark sky site can see as low as magnitude 6, a typical urban site can only do as dim as magnitude 4. A city area might be as high as 3, with only about 50 stars visible.
Next we learn about sky distances and the “celestial sphere”. We learn that we can estimate distances with our hands, with a finger being about 1 degree at arms length, fist being about 10 degrees, and your outstretched thumb-to-oustreteched pinky is about 20 degrees. The sun and moon are about 1/2 degree across, and the belt in Orion is about 3 degrees, and Rigel to Betelgeuse is about 18.5 degrees. Most people can only see about 1 arc minute resolution in the sky, and 1 arc second is about the size of a quarter at a distance of 3.1 miles away. Zenith is directly overhead, and he walks us through the definitions of true north (the rotational pole) and magnetic north, and how altitudes of stars and planets and their azimuths are determined, and alt-azimuth mounts for scopes. The meridian is explained as passing from the North Pole to the South Pole, passing directly overhead. The celestial equator is described as being 23 degrees above/below the horizon, to make up for the tilt in our orbital spin.
Next coordinate systems are explained, with the height above the horizon being Declination, and the E/W being Right Ascension, similar to latitude and longitude. These remain constant as the object moves across the sky, they pin the object to the celestial sphere, not our observing location. The declination is relative to the celestial equator (NOT the horizon, which is tilted). Right Ascension is zero on the vernal (spring-the Sun’s south to north crossing on March 20th) equinox point - where the ecliptic crosses the celestial equator. Since Orion’s Belt is near the celestial equator, it is a good way to locate it. The equator passes just below the belt at 5H 55m. He then describes the ecliptic and the zodiac, where the sun appears to traverse the heavens. Next he tells us that planets, unlike stars, don’t twinkle, and can so be verified in the night sky. He closes with a discussion of the Messier and NGC catalogs of sky numbers.
The naked eye is a powerful instrument—if you know how to use it. Learn the best times and conditions for observing, how to identify the positions and magnitudes of stars and planets, how the sky changes over the course of a night, how to use astronomical maps such as a planisphere, and more.
Episode 3 Using Binoculars and Backyard Telescopes 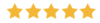
This episode was very good, but I knew most of this already. He starts out by covering how to purchase and use a good set of binoculars. He also makes the point that some objects, such as M45 the Pleiades star cluster, look better in binoculars because the are so visually large that a wide field of view (wider than a telescope allows) is required for good viewing. He shares that aperture (or objective in a telescope) is the light opening, and determines overall image brightness, and that magnification level is completely separate.
Next we learn how telescopes work, and how they typically don’t have prisms so the view is reversed left/right and upside down. We also learn about focal length, the distance down the tube where focus is achieved by the lens. He then shares that the eyepiece does the magnification, and that the objective determines the brightness again, and that generally more light collection is better.
Next we learn about types of telescopes.
Refactor - or types use lenses to focus light, generally at the end of a long tube. These are limited by the ability to grind big lenses, but also more importantly, the ability to support the sharp edges of a large lense under its own weight as we move it about the night sky. These typically suffer from achromatic aberrations, the fact that different wavelengths are bent different amounts. Some scopes do correct for this.
Reflectors - gather light on a large mirror in back of the tube that generally reflects back from the primary mirror up the tube to a smaller angled mirror that shines the light out the side to an eye piece such as the popular ”Newtonian” type scopes. In a Cassegrain reflector the light is sent back down the tube through a small hole in the primary mirror.
Catadioptrics - or combined scopes, with both mirrors and lenses.
Next he describes overall magnification as the focal length of the telescope over the focal length of the eye piece. Thus the shorter the eyepiece (6mm versus 50mm) the greater the magnification - but at a cost of smaller field of view. He tells us that most people use 2030x magnification per in of objective diameter when observing planets, and up to 60x on a good steady night. We can hardly ever use over 300x because of poor seeing. Next he reminds us that star twinkling is caused by unsteadiness in the intervening atmosphere. If the stars twinkle a lot the image in the telescope will be blurry.
Next we learn about field of view, or how much of the sky you can see in the eye piece. This usually means larger, more expensive lenses. Most eyepieces are quoted in apparent field of view. This is the field of view outside of a scope. The true field of view is the apparent FOV of the eye piece divided by the magnification. He then shares a cool tip. If you don’t know the FOV of the scope, find a star near the ecliptic. We know these stars move about 1 degree every 4 minutes, so if we place the star just outside the E edge of the scope and time how long it takes to clear the W edge of the scope and divide by 4, we’ll have the FOV.
Eye relief is how far away from the eyepiece you can still focus the lens. Most lenses can correct for near or farsightedness, but not astigmatism, so if you have astigmatism should buy eye pieces with large reliefs. Next he describes good start scopes as being very portable and easy to maintain. Since Refractors never need to be realigned, or collimated, and cannot get dust inside the lens system, they are easy to maintain and almost never need to be cleaned. A starter scope is 2-4” objective. Above 6” diameter is quite expensive. Achromatic scopes use multiple lens to get most of the usable light focused. Apochromatic scopes use several lens to fully correct for aberration.
Next he explains that Schmidt-Cassegrain and Muksutov-Cassegrain are some the most popular scopes. Then we move onto mount types, first alt-az mounts, which can cause it to be difficult to track stars. Then there is the equatorial mount that is aligned to the Earth’s rotational axis. A “GoTo” mount uses a computer to find and track objects in the sky. Equatorial mounts are really required for astrophotography, and some use “guiding” - a smaller scope that finds bright known objects and keeps them centered in the scope. Some scopes can use GPS to do their own basic alignments. Next we learn that standard eye pieces come in three sizes, .965”, 1.25”, and 2”, with the latter two being most common. They also come in focal lengths of 4mm-55mm (with the 4mm being very high magnification). It is rare that the air is steady enough (good seeing) to use more than 3-400x magnification - but also never than 60-80x per inch of aperture/objective. A ‘star diagonal’ brings the light out 90 degrees from the scope line and is really helpful for objects very close to the zenith. A Barlow lens increases the focal length, and therefore the magnification. A 2X Barlow doubles the focal length of the scope and doubles the magnification of any eyepiece that you put in the scope - it’s really important to buy a good Barlow. It also preservers the eye relief for those with glasses, so you can use a lower power eyepiece with a Barlow to get good magnification with a lower power eyepiece with good relief. We finish learning about filters. Filters thread into the bottom of standard eyepieces. First is the Moon filter that cuts the extreme brightness of the Moon when it’s near full. Some are adjustable even. Next is the light pollution filter, which dampens light in the manmade wavelengths, which is especially useful when looking at nebula.
here are many choices when selecting binoculars or a telescope. Learn what to look for in light-gathering power, optical design, magnification, mounts, and other features. Professor Murphy also suggests several tips for getting the best observing experience out of your equipment.
Episode 4 Observing the Moon and the Sun 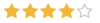
In this episode we first learn about how the Moon appears bigger when it’s on the horizon. Then we learn that the full moon always rises at sunset, which leads us to the Phases of the Moon. First we learn that “new moon” is completely black, directly to the sunward side of the Earth where we see the un-illuminated side. Then we find the crescent moon. It is 29.5 days from new moon to new moon. The first 2 weeks the moon rises at sunset, the next 2 it sets at sunrise. Waxing moons are lit from the right and waning at the left (in the northern hemisphere).
Next we learn that, although the moon rotates, the same side always faces the Earth (it is tidally locked), and the “dark” side looks very different than the side facing the Earth (which does actually get illuminated). This means it rotates on it’s axis in the time it takes to orbit the earth (once a month). Next we learn that it is best to view the moon near the quarter, and view along the ‘terminator’, or the day/night line, since the shadows bring out a lot of additional detail. We then learn that the highlands are 4.1-4.4 billion years old, and the maria (‘seas’, or the dark spots where molten rock filled in after heavy asteroids hit) are 3-3.8 billion years old. The Maria cover about 31% of the near side of the Moon. Then we learn that the craters about 10X bigger than the objects that created them. Next we learn that the “rays” are ejected material from impacts landing on the older, darker surface of the Moon so they appear brighter.
Next we learn about the Sun’s yearly trip through the stars of the Zodiac constellations. Then we learn that the seasons on Earth are related to our 23.5 degree tilt. In summer (in either hemisphere) that the tilt is towards the sun, and winter the tilt is away from the sun. The equinoxes are when the Sun crosses the equator, and the solstices are when the sun stops moving north or south, and starts heading the other way. The Tropics are mark where, on the solstices, the sun is directly overhead, and the sun is overhead of the equator on the equinoxes. Next we learn that the sun never rises within 23.5 degrees of the poles in mid winter, and never sets within that range in summer - the Arctic/Antarctic circle.
Charting the motions and changes of the sun and moon may be humankind's oldest astronomical activity. Discover how both objects offer rich opportunities for study. Also learn the precautions to take when observing the sun, which is the only star that can be seen up close and in detail.
Episode 5 Observing the Planets with a Telescope 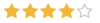
This episode starts with sharing that both Mercury and Venus both go through phases, just like the Moon does. Then we learn that there are 3 types of planets, the inner 4 (Mercury, Venus, Earth, and Mars) which are hard, rocky planets. Then we have the gas giants (Jupiter, Saturn, Uranus, and Neptune). Then we have the icy bodies, Pluto and Eris in the Kupier Belt.
Since Mercury is closer to the Sun than us, we can never see it very far from the Sun, so it is always seen close to dawn or dusk (best at it’s greatest elongation). The same is true of Venus, although it gets a little further away, but it is clearly larger when it is closer to us. Mercury is 38% the diameter of the Earth and 5.5% the mass, and orbits at .4 AU in 88 days. It has no atmosphere and looks muck like the Moon, but this is not visible from Earth with typical telescopes. It’s size varies from 5 to 13 arc seconds in the sky, depending on where it is in it’s orbit. Mercury is never more than 28 degrees from the Sun, so it is always low to the horizon, so trees and buildings, and turbulence are issues.
Venus is the hottest planet in the solar system, even though it’s twice as far away from the Sun as Mercury. It’s 95% the diameter of Earth and orbits in 225 days a .72 AU (so it gets closer to Earth than any other planet), and 82% the mass but the atmosphere is 92x that of Earth. The average surface temp is 870F. It’s as small as 10 arc second, and gets as big as 65 arc seconds on closest approach, and is the third brightest object in our sky. It reaches greatest elongation of 45-47 degrees from the sun, and is visible for many weeks in the sky.
Mars is one of the most popular objects to look at, but is difficult to see features on because it is so small, only 53% the diameter of the earth and 11% the mass, orbiting in 687 days (1.88 years) at 1.5 AU. To see detail on Mars it must be viewed at Opposition, when it’s closest to the Earth, which only happens every 26 months. Mars’ orbit the most elliptical orbit of the planets, so sometimes Mars is much closer (perihelic-56 million km) opposition, and much further away at aphelic (no closer than 101 million km) opposition. Can be as big as 25 arc seconds across at perihelic opposition, at aphelic it’s 14 arc seconds, and can be as small as 4 arc second when on the far side of the Sun. Viewing is good for a month on either side of opposition. Upcoming oppositions are October 13, 2020 and December 8 2022. Mars rotates every 24 hours and 40 minutes on its axis. The atmosphere is on .7% that of Earth, and mostly CO2. Mars has 2 small moon, Phobos (fear) and Deimos (panic) and are 22 and 12 km, respectively, and are probably captured satellites. Blue and green filters make the polar caps more visible, and red/yellow make the dust storms and dark patches more distinct. #15 deep yellow, #25 red, #58 green and #80a blue are common.
The gas giants are mostly hydrogen and helium. Jupiter is 11.2x the diameter and 318x the mass of the Earth (greater than the rest of the planets put together). It orbits in 12 years at 5.2 AU. At opposition Jupiter can be 44-50 arc seconds across, and never small than 30, and comes to opposition ever 13 months, and is good for about 2 months on either side of opposition. You’ll easily see the cloud bands and the 4 big moons (Is, Ganymede, Europa, and Callisto). Jupiter has dark belts and bright zones. She rotates in 9.8 hours. The red spot is 2-3x the size of the Earth. Io orbits in 1.8 days, Callisto requires 17 days.
Saturn is spectacular in a scope. It’s 9.5x the diameter and orbits 9.5 AU from Earth, and comes to opposition every 54 weeks when it is 18-21 arc seconds in size. It is well placed for a few months before and after opposition. The rings span more than 270,000 km, but are tens of meter thick. The rings are around the equator, but are tilted about 27 degrees with respect to the orbit. They appear edge on about every 15 years, and it orbits the sun about every 30 years. The rings are number A-C, with A being the outermost. Saturn has over 60 moons, the largest is Titan, and is the only moon in the solar system with a substantial atmosphere.
Uranus 4x diameter of the Earth and orbits at 19.2 AU in 84 years. At it’s largest it’s only 4 arc seconds across, and it’s axis is tipped 98 degrees to it’s orbital axis.
Neptune is a near twin to Uranus, and is 3.8x the diameter of Earth and orbits at 30AU, taking 165 years to orbit the Sun. At it’s best it’s only 2 arc seconds across.
Pluto is the largest of the Kuiper Belt Objects.
The rings of Saturn, the bands of Jupiter, the phases of Venus, the polar caps of Mars—these and other planetary features are visible through a small telescope. Learn when viewing opportunities arise for each of the planets and what sights await the dedicated observer." and…
Episode 6 Meteor Showers, Comets, Eclipses, and More 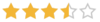
Interesting, but to me, not as interesting as some of the other episodes. First we learn that many meteor showers and comets are within traveling distance of us seeing, and that in fact there are often a couple of faint comets viewable with a small to medium telescope most of the time. Next we learn that comets orbit on highly elliptical orbits, often coming closer than Mercury and Venus to the Sun before heading back out into the solar system - and that most of the brightest have periods in the thousands of years! The comet tail can be millions of miles long, and always points away from the sun (due to the solar wind).
Meteor showers are caused when the Earth travels through debris left behind by a comet or asteroid (and minor planets). On any clear night you can expect to see a few to maybe 10 meteoroids per hour. They are meteoroids until they hit our atmosphere, at which point they become meteors, and anything that hits the ground is a meteorite. Most meteoroids are grain to pebble sized. They enter at 7-45 mi/sec, and the glow is caused by heated gas (air) around the meteor. A very bright meteor is a Fireball. The dense trail of meteoroids left by a comet can create meteor showers. These appear to come from one location in the sky called the Radiant, and are named for the constellation that they radiate from. A measure of how many meteors you will see in an hour is the Zenith Hourly rate, which is how many would see in an hour if they are near the zenith where there is little interference. All showers are generally 1 day before or after the published "best" day, depending on our orbit and your viewing location. A bright moon with obscure most meteors, and the field of view of a telescope or binoculars are too small to find meteors, and are typically best from midnight to noon, when you're looking in the direction of travel of the Earth's orbit.
Total eclipses, like comets, have long had negatively connotations in human history. It's a happy coincidence that the moon and the sun are both 1/2 degree across in our sky, so that total eclipses are possible. The umbra is the dark part of the shadow of the moon, and the penumbra is where it is not totally covered. There are ways to safely look at the sun, but must be strictly followed. Planets and bright stars appear in the sky, and there is light on all horizon directions. Next we’re told about annular eclipses, and how the Sun and Moon’s apparent sizes change over time, depending on how close they are, and times the Moon is simply not big enough to fully cover the sun. A total eclipse is at best 169 miles across and can last at most 7 minutes due to the moons speed (1,000 MPH).
Conversely we learn that total lunar eclipses are visible from the entire night side of the Earth, and do not happen every month because the Moon’s orbit is inclined at 5%. This inclination makes the Moon miss the Earth’s umbra on most full moons. They only happen twice a year, during ‘eclipse season’ when the Moon is passing from above or below the ecliptic to the other side, just as the sun does with changing seasons. This is where the ecliptic gets its name. Next we learn about penumbra, partial, and total eclipses. A total eclipse of the Moon can last for 2 hours, so in 3-4 hours we go from full moon to partial then full eclipse, and back to full. Because light bends around the Earth usually the eclipsed Moon looks reddish. There are 2-4 lunar eclipses every year, with a total eclipse every 18 months or so.
Finally we learn that Mercury (7%) and Venus (3.4%) also cast shadows onto Sun. Mercury requires a telescope. This happens 13 or 14 times a century. Transit of Venus happen in pairs, separated by 8 years, but the transits only happen every 105.5 or 121.5 years, in January and June and last about 6 hours. The next topic are halos around the moon or sun, which are high cirrus clouds filled with hexagonal ice crystals, and the light gets bent by 22 degrees or more creating the halo (sun dogs). Then we learn that satellites can been seen a few hours after sunset or before sunrise, when they are still above the horizon and in the sun.
Explore a variety of special phenomena that are among the wonders of the sky. Some, like bright meteors, aurora, and many comets, are largely unpredictable. Others, like eclipses and annual meteor showers, occur at well-known times—although it may require a special trip to see them.
Episode 7 The Northern Sky and the North Celestial Pole 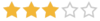
This episode starts out with Ursa Major, which includes the Big Dipper. We learn that the two stars at the end of the dipper point us to the North Star Polaris (28 degrees away, a little further than your outstretched fingers plus your fist) - and it’s the end star of the Little Dipper. The W of the Cassiopeia can be found by drawing a line from the Big Dipper through Polaris to the middle star of Cassiopeia, which lies int he Milky Way. The stars appear to move on a ‘celestial sphere’, and the stars appear to rotate counterclockwise. Next we learn about Southern Hemisphere stars that never rise for mid northern latitudes like the US and Europe, such as the Southern Cross. You never see any part of the cross above 33 degrees, and you must be below 27 degrees to see all of the cross. Next we learn that the altitude of Polaris is the same as our latitude.
Next we learn about the wobble of the Earth, or precession, which takes about 26,000 years. This means that the north celestial pole moves through Vega and Thuban. Since Right Ascension is defined as zero where the vernal equinox happens in the stars of the zodiac, and this is moving with precession, star catalogs must publish the year, or epoch, that they are good for as the RA is constantly changing.
Embarking on the second half of the course in which you systematically tour the entire sky, study two constellations that are continuously in view from the Northern Hemisphere: Ursa Major and Cassiopeia. Also explore the slowly shifting position of true north in the sky.
Episode 8 The Fall Sky 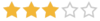
Again, interesting, although I’m not a huge fan of the ‘sky tour’ episodes. As such I’m not going to retell the constellation myths, but they are entertaining. We do learn about binary star pairs and how exoplanets are discovered, such as the 3 around upsilon Andromeda. Then we learn about the Andromeda galaxy at 2.5 million light years away it is the furthest object you can see with the naked eye. It contains about 400 billion stars, nearly double the Milky Way although we nearly as massive, and is the closest example of spiral galaxy. It’s tipped about 77 degrees to our line of sight, so it’s nearly edge on. It’s 150,000 LY across, about 1.5 times the Milky Way. Andromeda is the largest in our local group (the Milky Way, M31 Andromeda, M33, and about 30 smaller ones). Andromeda is approaching at 75 miles/sec, and we expect them to collide in about 3 billion years. M31, the Andromeda galaxy, is 8-10 times the length of the diameter of the full moon. M31 has 2 companions, M110 and M32, both dwarf elliptical galaxies.
Just south of Andromeda is the Triangulum, which features M33, the Triangulum Galaxy which is the smallest of our 3 spiral galaxies in our Local Group, but we see it face on and is often called the Pinwheel galaxy. It is 1/2 the diameter and 1/7 the mass of the Milky Way.
Navigate your way around the autumn sky from the Northern Hemisphere, discovering how the classical myth of Andromeda ties together the stories of the nearby constellations of Cassiopeia, Perseus, Cepheus, Pegasus, and Cetus. The sights include the Andromeda galaxy, the nearest large galaxy to our own.
Episode 9 The Winter Sky 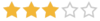
Another pretty good episode, we start by learning about Orion and the constellations he can point us to. Next we learn about Betelgeuse in Orion’s armpit, a Class M super giant star, 640 LY away from our Sun, and is the biggest star of any kind within a few thousand light years of the sun. If it were in place of our sun, it’s radius of 5AU would consume all of the inner solar system and out to Jupiter. It is one of the very few stars that are close enough that we can see surface features. It is a red giant that is beginning to die, having used up the majority of it’s hydrogen. When it dies it will be brighter than the Moon and visible in the daytime for a few months. In the belt is Orion’s Nebula, which looks like a fuzzy greenish white cloud with 4 new born stars (the Trapezium) buried within. The nebula is 1530 LY away, making it the closest large star forming region (all these stars are under 1 million years old). Also on Orion’s Belt is the Horsehead nebula, a smaller nebulosity.
Canis Major and Minor, Orion’s hunting dogs, including Sirius - a A1 class star only 8.6 LY away, one of the closest star systems, and the brightest in our skies. Next we move to Taurus the Bull and the Pleiades. Aldebaran is the largest star in Taurus, which means ‘the follower’ because it follows Taurus. It’s a K3 only 67 LY from the Sun.
Open Clusters typically have 50-100 stars in the plain of the Milky Way with young, hot, blue stars. Globular clusters are bigger, having a 10,000 to a million and are not on the plane, but typically surrounding it in a halo near the center. All the stars in a cluster are the same distance away from us, and have the same composition. The Pleiades (M45) is an open cluster, ‘the Seven Sisters’. Folklore has it that it’s time to plant when the Sister become visible, and to harvest when the set for the winter.Between Perseus and Cassiopeia is the double cluster NGC 884/869, the open clusters. A low power, wide field eye piece, will fit both clusters. Another nearby cluster are the Hyades within the face of the Bull. The Hyades are one of the most clusters for judging distances and luminosities of other stars, and are just 151 LY away. Also in Taurus is M1, the Crab Nebula, and exploded star.
Next we learn about Castor and Pollux, the heads of the Gemini twins. Near the twins feet is M35, an open cluster. In Cancer we find M44, the Beehive cluster (or Praesepe). The Tropic of Cancer was named for this constellation as the Sun used to be in Cancer on the solstice, but precession has since moved it. The Beehive is a good binocular target, about 550 LY away, with about 1,500 stars about 600 million years old. Within Auriga the charioteer is Capella, but it contains M36/37/M38, all good small telescope objects.
Continuing your focus on the constellations of the Northern Hemisphere, survey the magnificent winter sky, dominated by Orion. "Star hop" around the region, which includes a wealth of interesting stars, globular clusters, nebulae, and other features, especially the Orion Nebula—the finest nebula in the northern sky—and the Pleiades and Hyades star clusters in Taurus.
Episode 10 The Spring Sky 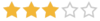
Pretty good, but constellation history is covered everywhere, so a lot of this wasn't really new to me. Ursa Major, Virgo, and Bootes are the main constellations. Leo is also in the sky, and Leo contains Regulus about 79 LY away, and Algieba which is a double star (126 LY away). Leo also contains 2 triplets of galaxies about 26 million LY away M95/96/105 is the first and M65/66/NGC3628 the second.
There is in this part of the sky, near Virgo, is the Realm of galaxies. In the diamond of Virgo, between Arcturus, Cor Carolina, Denebola, and Spica, on the right hand side between Virgo and Coma Berenice are a hundred or more galaxies, about 60 million light years away and 14 million wide, including M84/86/87. Many of these are elliptical galaxies which only have old yellow, orange, and red stars, having used up their gas long ago. Within M87 is a supermassive black hole with a large accretion disk and large jets of material being expelled close to the speed of light. This makes it (Virgo A) one of the brightest radio targets.
In the southern part of Virgo is a nearly edge-on spiral galaxy, M104 the Sombrero Galaxy.
The spring sky opens the view into intergalactic space perpendicular to the plane of the Milky Way. Among the objects visible are the immensely rich galaxy clusters in Virgo and Coma Berenices, which are many millions of light-years distant and can be seen with small and moderate telescopes.
Episode 11 The Summer Sky 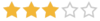
Pretty good, but again, a sky tour. We start by learning how to use Ursa Major to find the Summer Triangle and Vega (and the Northern Cross). Then we learn about Epislon Lyrae, the double double star. Apparently those with excellent vision can detect this is a double star, but with about 100X-125X magnification shows that each star is actually a double. Then ere is the Ring Nebula (M57), which requires a scope, but with 125-200X magnification you can see what looks like a smoke ring. This is a planetary nebula, the final stage of a star, much lower power than a supernova, and this is what we expect to happen to the Sun.
At the top of the Summer Triangle is Deneb, 1,425 LY away, a blue super giant 110x the radius and 54,000X more luminous than the Sun and in one night it puts out as much energy as the sun in 300 years. Then we look at Albiero, the most beautiful double in the sky with a 3.1 yellow K giant and 5.1 hot blue B8 star 34 arc seconds apart (pretty far for binaries). Within Hercules is M13, the great globular cluster, about 25,000 LY away 150 LY across, and contains about few hundred thousand stars. Also in Hercules is M92 is another globular cluster in the northern part of the constellations.
Near Antares in Scorpius is the globular cluster M4, too close to Antares to see with the naked eye. Also in Scorpius are M6 (the Butterfly) and M7 (Ptolemy’s cluster), open clusters. M6 has about 50 stars, M7 appears as a bright knot, and is best viewed with binoculars. Just outside of Scorpius is 18 Scorpii, a star very similar to our Sun, 45.7 LY away.
Ophiuchus is a huge constellations to the north of Scorpius. Nearby is Sagittarius, which is on the plane of the Milky Way, so it is very rich, but it does not rise very high in mid northern latitudes. Here is M8, the Lagoon Nebula, which looks split in half by a lagoon. Just north and west is M20, the Trifid nebula. Also M17 (Omega or Swan) and M16 (Eagle nebula) are here.
Arching high overhead in the summer sky is the Milky Way, which is the plane of our galaxy seen from the inside. Tour this densely packed region of stars of all types, from dusty regions of star birth to the exquisite shells of dying stars. Here, a useful orienting feature is the Summer Triangle.
Episode 12 The Southern Sky and the Milky Way 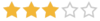
A sky tour and of a sky I’ll almost never see. Meh. Not that it isn’t well done, but just not much use to me. First we learn about Crux, the Southern Cross, not to be confused with the false cross. It is on the flags of Australia, New Zealand, Papua New Guinea, and Brazil. Acrux is the brightest star at the bottom of the cross. The coal sack nebula is just south and west of the Cross in the Milky Way.
Alpha Centauri is the third brightest star in the sky, and is in Centaurus, and is 4.36 LY away, and is the closest star system to our Sun, and is a binary. A is a G2 Star just like our sun, and the partner is a K2 cooler star. Also nearby is Proxima Centauri, only 4.22 LY, the closest of all. It also contains the globular Cluster Omega Centauri. Also the galaxy Centaurus A, NGC5128, visible in binoculars with a dark dust plane, but otherwise looks like an elliptical.
Near the Small Magellanic Cloud is 47 Tuncanae, a globular cluster. In late spring and early summer the large Magallanic cloud are both visible, companion galaxies to our Milky Way. They are both irregular galaxies. The tarantula nebula is also nearby. Canopus, or Alpha Carinae, is in the Argo nearly due south of Sirius and is a rare Class F star. The Carina Nebula is nearby at 75 LY and is 4X larger than the Orion Nebula. In here is Eta Carina, which is a huge and unstable star.
In this final lecture, travel to the Southern Hemisphere for sky views inaccessible from northern latitudes. Discover the famous Southern Cross, the Large and Small Magellanic Clouds, and a spectacular panorama of the Milky Way—along with new myths and stories that add a human dimension to our marvelous night sky.